New battery technology breakthrough is poised to reshape industries from transportation to consumer electronics. This exciting advancement promises greater energy density, longer lifespans, and enhanced safety features. The evolution of battery technology, from early prototypes to modern innovations, has been remarkable, and this progress is only accelerating.
This overview explores the key aspects of this breakthrough, examining various chemistries, manufacturing processes, and the impact on different sectors. We’ll delve into the challenges, safety considerations, and the potential future of battery technology.
Introduction to New Battery Technology Breakthroughs
Recent advancements in battery technology represent a significant leap forward, pushing the boundaries of energy storage capabilities. These breakthroughs are poised to revolutionize various sectors, from transportation and consumer electronics to large-scale energy storage systems. The implications are far-reaching, promising increased efficiency, longer lifespans, and greater safety for devices and systems reliant on power.These advancements are fueled by a deeper understanding of electrochemical processes and the development of novel materials and chemistries.
This understanding allows engineers to design batteries with superior performance characteristics, ultimately benefiting diverse applications. The historical trajectory of battery technology provides a crucial context for appreciating the magnitude of these recent innovations.
Definition of New Battery Technology Breakthroughs
New battery technology breakthroughs encompass innovations in battery design, materials, and manufacturing processes that result in demonstrably improved performance metrics compared to existing technologies. These improvements encompass factors such as energy density, power density, cycle life, safety, and cost-effectiveness.
Significance of Advancements in Various Sectors
The impact of these advancements is substantial across multiple sectors. In transportation, increased energy density translates to longer driving ranges for electric vehicles (EVs) and hybrid vehicles. In consumer electronics, improved battery life extends the operational time of smartphones, laptops, and other portable devices. Large-scale energy storage solutions benefit from higher energy storage capacity and longer lifespan, crucial for grid stabilization and renewable energy integration.
Historical Context of Battery Technology
Battery technology has evolved significantly over the past two centuries. Key milestones include the invention of the voltaic pile in the late 18th century, which marked the beginning of electrochemical energy storage. The development of the lead-acid battery in the 19th century revolutionized transportation and industrial applications. The emergence of lithium-ion batteries in the late 20th century ushered in a new era of portable electronics and electric vehicles, demonstrating a notable advancement over previous technologies.
Examples of Innovative Battery Chemistries and Materials
A wide array of innovative battery chemistries and materials are currently being explored. One example is the use of solid-state electrolytes in place of liquid electrolytes, promising enhanced safety and higher energy density. Furthermore, research into new cathode materials, such as lithium-metal oxides and sulfides, is continually producing promising results. These advanced materials and chemistries are crucial for the next generation of high-performance batteries.
- Solid-state batteries utilize solid electrolytes, offering improved safety and potentially higher energy densities compared to traditional liquid-electrolyte batteries. This advancement mitigates the risks associated with liquid electrolytes, such as leakage and thermal runaway.
- Lithium-sulfur batteries offer a high theoretical energy density, surpassing that of lithium-ion batteries. However, challenges remain in achieving practical cycle life and addressing material compatibility issues.
- Lithium-air batteries hold the potential for extremely high energy densities, potentially revolutionizing energy storage. However, significant research is still needed to overcome challenges related to catalyst stability and the development of efficient air electrodes.
Technological Advancements
Recent breakthroughs in battery technology are poised to revolutionize various sectors, from electric vehicles to portable electronics. These advancements encompass a spectrum of improvements, from enhanced battery chemistries to more sustainable manufacturing processes. This section delves into the specifics of these advancements, highlighting the key improvements and potential impacts.The evolution of battery technology is a crucial aspect of our pursuit of a sustainable future.
Greater energy storage capacity and faster charging times are driving innovations in electric vehicle range and charging infrastructure, while advancements in portable electronics offer longer lasting devices.
Different Battery Chemistries
Various battery chemistries are currently under development or in use, each with its unique strengths and weaknesses. Lithium-ion batteries, the dominant technology in portable electronics and electric vehicles, are known for their high energy density, enabling devices with long operational times. However, their use of flammable electrolytes presents safety concerns.Solid-state batteries, an emerging technology, aim to overcome these safety limitations by using solid electrolytes.
These electrolytes are generally safer and offer the potential for higher energy densities and faster charging times than conventional lithium-ion batteries. Examples of applications include electric vehicles and portable electronics.Flow batteries, a different approach, utilize liquid electrolytes in separate tanks. While their energy density may be lower than lithium-ion batteries, flow batteries can be scaled to larger capacities, making them suitable for stationary energy storage systems, such as grid-scale energy storage.
This makes them particularly attractive for renewable energy integration.
Performance Characteristics Comparison
Battery performance is evaluated based on several key metrics. Energy density measures the amount of energy a battery can store per unit of mass or volume, directly impacting the range of electric vehicles or the operational time of portable devices. Power density, conversely, indicates how quickly a battery can deliver power, influencing the acceleration capabilities of electric vehicles or the response time of electronic devices.
Finally, lifespan is a critical factor, representing the duration a battery can maintain its performance before requiring replacement.
Battery Type | Energy Density (Wh/kg) | Power Density (W/kg) | Lifespan (cycles) |
---|---|---|---|
Lithium-ion | 100-250 | 500-1500 | 500-1000 |
Solid-state | >250 (projected) | >1500 (projected) | >1000 (projected) |
Flow | 50-150 | 100-500 | >5000 |
Advancements in Manufacturing Processes
Sustainable and scalable manufacturing processes are crucial for the widespread adoption of next-generation batteries. Researchers are exploring techniques to reduce material waste, minimize the use of hazardous substances, and enhance the efficiency of production lines. This includes employing recycled materials and developing new, more sustainable manufacturing methods.
Next-Generation Battery Materials
The development of novel materials is pivotal in enhancing battery performance. New materials with improved conductivity, stability, and safety properties are being investigated. For instance, advancements in electrode materials can significantly boost energy density, while novel electrolyte materials enhance safety and stability. The use of advanced materials and improved manufacturing processes aims to ensure the long-term viability of these technologies.
“The development of sustainable and scalable manufacturing processes is a key element in the widespread adoption of next-generation batteries.”
Impact on Industries: New Battery Technology Breakthrough
This groundbreaking battery technology promises a profound impact across numerous industries, particularly in transportation, energy storage, and consumer electronics. Its enhanced performance characteristics, including increased energy density and faster charging times, offer significant advantages compared to existing solutions. These improvements will translate into tangible benefits for consumers and businesses alike.
Transportation Sector
This revolutionary battery technology is poised to reshape the transportation landscape, particularly for electric vehicles (EVs) and autonomous vehicles. The increased energy density will allow EVs to travel significantly longer distances on a single charge, reducing range anxiety and expanding their usability. This will also accelerate the adoption of EVs, potentially leading to a more sustainable transportation system.
- Enhanced Performance for EVs: Higher energy density directly translates into extended driving ranges for electric vehicles. This allows for more extensive commutes and reduces the need for frequent charging, making EVs more convenient and practical for daily use. This increased range addresses a significant barrier to EV adoption, making them a more viable option for long-distance travel.
- Advancements in Autonomous Vehicles: The improved battery technology’s compact size and higher power output will contribute to the development of smaller, more efficient autonomous vehicles. The reliability and performance characteristics of these batteries will contribute significantly to the success and widespread adoption of autonomous vehicles, potentially revolutionizing transportation.
Energy Storage Solutions
The potential of this advanced battery technology extends beyond the transportation sector, impacting residential and commercial energy storage solutions. Homes and businesses can benefit from greater energy independence and resilience by storing renewable energy sources like solar and wind power. This can reduce reliance on the grid and provide a more stable and sustainable energy supply.
- Residential Energy Storage: These batteries can be integrated into homes, enabling homeowners to store excess solar energy for later use. This feature allows for greater energy independence and cost savings, potentially reducing reliance on expensive utility bills.
- Commercial Energy Storage: Businesses can leverage these batteries to store energy generated from renewable sources. This can result in substantial cost savings and a more reliable energy supply, minimizing downtime and enhancing operational efficiency.
Consumer Electronics
The enhanced battery technology is expected to revolutionize the consumer electronics market, leading to significant improvements in portable devices and wearable technology. Increased energy density will translate to longer battery life, while faster charging times will provide a more convenient user experience.
- Extended Battery Life for Portable Devices: Portable devices like smartphones, tablets, and laptops will experience significantly longer battery life, reducing the frequency of charging and improving user productivity.
- Improved Wearable Technology: The improved battery technology will empower wearable devices like smartwatches and fitness trackers to operate for longer periods without needing frequent charging. This will enhance their utility and adoption by users.
Renewable Energy Support
The advancements in battery technology play a crucial role in supporting the growth and integration of renewable energy sources. The enhanced capacity and performance characteristics of these batteries will allow for efficient storage and management of intermittent renewable energy sources like solar and wind power. This enables the transition towards a more sustainable energy system.
- Grid Stability: The technology’s ability to store and manage fluctuating energy from renewable sources will improve the stability and reliability of the electricity grid.
- Facilitating Renewable Energy Integration: The enhanced capacity and efficiency of these batteries will facilitate the widespread integration of renewable energy sources into the existing energy infrastructure.
Challenges and Considerations
The revolutionary advancements in battery technology present exciting opportunities but also pose significant hurdles. Navigating these challenges is crucial for widespread adoption and realizing the full potential of these innovations. Addressing safety, environmental, and economic concerns is paramount to ensuring a smooth transition and a sustainable future for battery technology.
Safety Concerns
Battery safety is paramount. New chemistries and designs, while potentially offering superior performance, may introduce inherent safety risks. Thermal runaway, a rapid and uncontrolled increase in temperature, is a critical concern, especially with high-energy density batteries. This can lead to fires and explosions, necessitating robust safety mechanisms in battery design, manufacturing, and end-user applications. Rigorous testing protocols and safety certifications are essential to mitigate these risks and ensure public safety.
Environmental Impact
The environmental impact of battery materials and manufacturing processes is a growing concern. The extraction of certain battery materials, like lithium, can have significant environmental consequences, including habitat destruction and water pollution. Furthermore, the manufacturing processes can generate substantial waste and release harmful pollutants into the atmosphere. Sustainable sourcing practices and environmentally friendly manufacturing methods are crucial for mitigating the negative environmental impact of battery production and use.
The lifecycle assessment of batteries, from raw material extraction to end-of-life management, needs careful consideration to ensure a responsible approach to battery technology.
Economic Considerations
The economic viability of new battery technologies is a significant factor in their adoption. Production costs are a critical consideration. The cost of raw materials, manufacturing processes, and scaling up production significantly impact the overall price of batteries. The initial cost of new technologies often outweighs the advantages of performance and sustainability, hindering broader market penetration. A critical assessment of production costs and potential economies of scale is needed to ensure cost-effectiveness.
Market potential and demand forecasting are also essential elements. The size and growth of potential markets for new battery technologies determine the long-term economic viability of the industry. Government incentives and policies, including tax credits and subsidies, can stimulate the market for these innovative technologies.
Production Costs and Market Potential
- Raw Material Costs: Fluctuations in the price of critical raw materials, such as lithium and cobalt, directly influence battery production costs. The availability and accessibility of these materials are crucial for long-term sustainability and cost stability.
- Manufacturing Processes: Optimizing manufacturing processes is essential for reducing production costs and improving efficiency. Advanced manufacturing techniques and automation play a crucial role in scaling up production while maintaining quality control. Research and development focused on streamlining manufacturing processes can lead to substantial cost reductions.
- Market Demand: The projected demand for new battery technologies is a critical indicator of market potential. Understanding consumer preferences and industry needs will help determine the size and growth trajectory of the market. Forecasting future demand, considering technological advancements and consumer adoption rates, is crucial for investment decisions and market strategy.
Research and Development
Ongoing research into advanced battery technologies is crucial for driving innovation and ensuring a sustainable future. Significant efforts are being invested in improving energy density, charging speed, lifespan, and safety, which directly impacts various sectors. These advancements are fueled by a global commitment to developing more efficient and reliable power sources.
Ongoing Research Initiatives
Research initiatives are focused on multiple fronts to enhance battery performance and safety. These efforts involve exploring new materials, optimizing manufacturing processes, and developing advanced battery management systems. A key aspect of this research is the exploration of novel electrode materials, electrolyte compositions, and cell architectures to increase energy density and power output.
Key Players and Institutions
Numerous institutions and companies are actively engaged in battery research and development. Leading universities, national laboratories, and major battery manufacturers are at the forefront of this endeavor. For instance, renowned universities like MIT, Stanford, and Caltech, alongside research institutions such as the National Renewable Energy Laboratory (NREL), are conducting cutting-edge research. Private sector players, such as Tesla, LG Chem, and Panasonic, are also heavily invested in advancing battery technology to meet their specific industry needs.
Funding Mechanisms
Government grants and funding initiatives play a vital role in supporting battery research and development. Private sector investments, particularly from companies seeking to advance their products, are also substantial. Government agencies, recognizing the strategic importance of this technology, allocate funds for research through various programs focused on energy storage. For instance, the U.S. Department of Energy’s Office of Energy Efficiency and Renewable Energy provides substantial funding for battery research and development projects.
Venture capital firms are also increasingly interested in investing in promising battery startups.
Potential Future Directions
Future research will likely focus on developing more sustainable and cost-effective battery chemistries. The development of solid-state batteries is a promising area of research. These batteries, with their potential for higher energy density and improved safety, are expected to revolutionize the energy storage landscape. Further research into advanced battery management systems will also be crucial to optimize battery performance and safety.
The pursuit of flexible and 3D battery designs, along with the development of advanced charging technologies, are other potential avenues for future advancements. These efforts will be guided by the need to create more sustainable and accessible energy solutions for various applications.
Applications and Use Cases
This groundbreaking battery technology presents exciting opportunities across diverse sectors, from powering advanced vehicles to revolutionizing industrial processes. Its enhanced performance characteristics, including extended lifespan and higher energy density, pave the way for innovative applications. This section details specific use cases and analyzes the potential advantages and drawbacks of deploying this new technology in various industries.
Aerospace Applications
This advanced battery technology offers significant advantages for aerospace applications, particularly for electric aircraft and satellites. Its high energy density and exceptional power output translate into extended flight durations and enhanced payload capacities for electric aircraft. Satellites can benefit from the increased operational lifespan and reduced maintenance needs provided by these long-lasting batteries.
- Electric Aircraft: The technology allows for longer flight ranges, reduced weight compared to current lithium-ion batteries, and the potential for autonomous flight, crucial for drone delivery systems and personal air travel. Reduced weight allows for larger payloads or extended flight times.
- Satellite Propulsion: The advanced battery design facilitates prolonged satellite operation without frequent recharging or replacement. This translates to extended observation periods and reduced costs associated with maintenance and launch frequency.
Marine Applications
The marine sector can leverage this new battery technology for a variety of applications. The robust design and high energy density of these batteries make them suitable for powering electric ships and marine vessels, improving efficiency and reducing environmental impact. Their enhanced safety features are crucial for marine environments, reducing the risk of accidents.
Recent breakthroughs in battery technology are really exciting, promising significant advancements in portable electronics. This progress aligns nicely with the broader trends in Integrated Digital Technologies A Comprehensive Overview, Integrated Digital Technologies A Comprehensive Overview , showcasing how integrated systems are crucial for realizing the full potential of these new batteries. Ultimately, these advancements in battery tech will fuel the next generation of innovative digital devices.
- Electric Ships: This technology enables longer voyages without refueling, and reduces emissions, aligning with growing environmental regulations. The high power density allows for rapid acceleration and maneuverability.
- Autonomous Underwater Vehicles (AUVs): Extended battery life allows for longer underwater exploration missions without the need for frequent recharging or resupply, expanding the scope of underwater exploration.
Industrial Applications
The industrial sector stands to gain significantly from this innovative battery technology. Its reliable performance and durability make it suitable for a variety of industrial applications, from powering heavy machinery to supporting mobile robots in manufacturing. The adaptability of the technology allows for customized solutions for various tasks.
- Heavy Machinery: This technology can power construction equipment, mining vehicles, and other heavy machinery, improving productivity and efficiency while minimizing noise and emissions.
- Mobile Robots in Manufacturing: These batteries can power mobile robots, facilitating automated material handling, assembly, and inspection processes within factories. Their adaptability ensures they can be integrated into various existing systems.
Table: Advantages and Disadvantages of Use Cases
Use Case | Advantages | Disadvantages |
---|---|---|
Electric Aircraft | Extended flight range, reduced weight, potential for autonomous flight | High initial cost, regulatory hurdles, safety concerns |
Satellite Propulsion | Extended operational lifespan, reduced maintenance, lower launch costs | Potential for limited power output, complexity of integration |
Electric Ships | Reduced emissions, extended voyage duration, increased efficiency | High upfront cost, potential impact on existing infrastructure |
AUVs | Extended underwater exploration, reduced recharging needs, enhanced mission duration | Potential for limited depth capabilities, challenges in harsh underwater conditions |
Heavy Machinery | Improved productivity, reduced emissions, enhanced efficiency | Higher initial cost, potential for complex integration, specialized maintenance needs |
Mobile Robots in Manufacturing | Automation of processes, improved accuracy, enhanced safety | Potential for cybersecurity vulnerabilities, reliance on consistent power supply |
Safety and Reliability
Ensuring the safety and reliability of new battery technologies is paramount. The potential for catastrophic failures, such as fires or explosions, necessitates rigorous design and testing protocols. A robust safety framework is crucial to prevent accidents and build consumer confidence in these innovative energy storage solutions.
Safety Measures in New Battery Designs
Advanced battery designs incorporate multiple safety mechanisms to mitigate risks associated with overheating, short circuits, and other potential hazards. These include advanced thermal management systems, incorporating sophisticated cooling mechanisms and heat dissipation materials. Furthermore, advanced separators and protective coatings prevent short circuits by isolating the electrodes. Safety interlocks and fuse mechanisms are also strategically placed to immediately disconnect the battery in case of critical events.
Testing Procedures for Evaluating Battery Safety and Reliability
Comprehensive testing protocols are employed to assess the safety and reliability of new battery designs. These protocols include rigorous thermal runaway tests, simulating extreme conditions to determine a battery’s response to overheating. Short circuit tests, which involve applying high current loads to identify and measure the response of the battery’s protection mechanisms, are also crucial. Furthermore, mechanical stress tests evaluate the battery’s ability to withstand physical impact and vibration.
The results of these tests are meticulously analyzed to identify any potential failure points and improve the battery design.
Ensuring Long-Term Battery Performance and Durability
Long-term battery performance and durability are ensured through several strategies. Employing advanced materials with enhanced chemical stability and resistance to degradation is critical. Furthermore, sophisticated monitoring systems and predictive maintenance algorithms allow for early detection of potential issues, enabling proactive intervention to maintain peak performance. Optimized manufacturing processes, including quality control measures, guarantee consistent battery quality and minimize variability.
Standards and Regulations Governing Battery Technologies
Stringent standards and regulations govern the production and use of new battery technologies. These standards Artikel the safety requirements for battery design, manufacturing, and disposal. For instance, they define acceptable levels of thermal runaway, short circuit current, and mechanical stress resistance. Compliance with these regulations is crucial for both manufacturers and users to ensure safety and prevent potential harm.
Furthermore, specific safety guidelines are in place for handling, transporting, and storing batteries to minimize risks during use.
Manufacturing and Supply Chain
The successful commercialization of any new battery technology hinges critically on its manufacturability and the efficiency of its supply chain. This section delves into the intricacies of manufacturing processes for various battery types, the steps involved in the supply chain, key players, and the challenges associated with scaling production.The complexity of battery production necessitates meticulous control over every stage, from sourcing raw materials to final quality assurance.
This attention to detail is crucial to ensuring both cost-effectiveness and the desired performance characteristics.
Manufacturing Processes for Different Battery Types
The manufacturing process for lithium-ion batteries, the dominant type in current use, involves several key steps, including the preparation of cathode and anode materials, the assembly of the battery cells, and the packaging of the finished products. Different chemistries have slightly varying procedures, however, all follow a general pattern. For instance, lithium-nickel-manganese-cobalt (NMC) cathode materials require precise mixing and calcination processes, whereas lithium iron phosphate (LFP) cathodes have a simpler production process.
- Lithium-ion Batteries: The manufacturing of lithium-ion batteries involves the fabrication of electrodes (cathode and anode), the assembly of the battery cell, and the integration of safety features. These steps often involve sophisticated equipment and processes to ensure precise control over material properties, resulting in high-performance and reliable batteries.
- Solid-State Batteries: The production of solid-state batteries is still under development. Early prototypes demonstrate promising characteristics, but the production processes are still evolving and are expected to face challenges in terms of scalability and cost-effectiveness. The development of suitable solid electrolytes and the precise manufacturing of the solid-state electrodes will be critical to successful implementation.
- Other Battery Types: Other battery types, such as flow batteries and fuel cells, have distinct manufacturing processes. Flow batteries typically involve the fabrication of tanks and pumps to manage the flow of electrolyte solutions. Fuel cells require the production of electrodes and the assembly of the electrochemical components.
Supply Chain for Raw Materials, Components, and Finished Products, New battery technology breakthrough
The supply chain for battery materials and components is intricate and global. Raw materials like lithium, cobalt, and nickel are often sourced from geographically dispersed regions. The production of cathode and anode materials involves various intermediate steps, creating a complex web of suppliers and manufacturers.
- Raw Material Sourcing: The sourcing of critical raw materials, like lithium, is vital to the production of batteries. Geopolitical factors and environmental concerns can impact the availability and cost of these materials, making diversification of supply sources a crucial aspect of the supply chain.
- Component Production: The manufacturing of components like separators, current collectors, and packaging materials requires specialized expertise and infrastructure. Quality control throughout these stages is paramount.
- Final Product Assembly: The assembly of finished battery packs requires meticulous attention to detail. This process often involves sophisticated robotic systems and quality assurance measures to ensure consistency and safety.
Key Players in Manufacturing and Supply Chain
The battery manufacturing and supply chain involves a diverse range of companies. Large battery manufacturers are often integrated with component suppliers, creating vertical integration. Specialized materials producers and equipment manufacturers play a vital role in the overall ecosystem. Government policies and regulations also significantly impact the landscape.
- Battery Manufacturers: Companies specializing in battery production are essential for turning raw materials and components into finished products. Their scale and efficiency directly affect the final cost and availability of batteries.
- Material Suppliers: The mining and processing of raw materials, such as lithium and cobalt, are essential steps in the supply chain. Sustainability concerns are increasingly driving these companies to adopt environmentally friendly practices.
- Component Suppliers: Companies specializing in the production of battery components like separators and electrolytes are integral to the overall production process. Innovation in these areas is crucial to improve performance and safety.
Challenges in Scaling Up Production
Scaling up production to meet growing market demand presents several significant challenges. Ensuring consistent supply of raw materials, addressing production bottlenecks, and maintaining quality control are key hurdles.
- Raw Material Availability: The availability of key raw materials like lithium is often insufficient to meet growing demand. Geopolitical instability and environmental regulations can further complicate the situation.
- Production Capacity: Expanding manufacturing capacity to meet the rapidly increasing demand for batteries can be challenging, requiring significant investment in new facilities and equipment.
- Supply Chain Disruptions: Disruptions in the supply chain, whether due to geopolitical events or natural disasters, can create significant bottlenecks and impact the timely delivery of batteries.
Future Trends
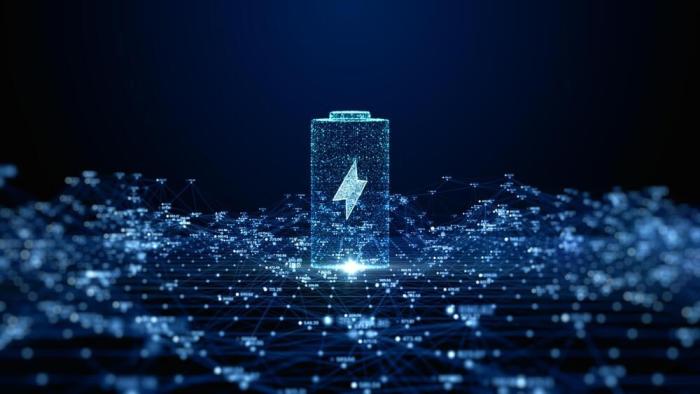
The future of battery technology is poised for rapid advancement, promising significant improvements in energy storage capacity, efficiency, and cost-effectiveness. This evolution will reshape various industries, from electric vehicles to renewable energy storage, driving a global transition towards sustainable energy solutions. Predicting the exact trajectory is challenging, but analyzing current trends and anticipated technological breakthroughs offers valuable insights into the likely future landscape.
Projected Market Growth
The global battery market is experiencing substantial growth, driven by the increasing demand for electric vehicles and energy storage systems. Analysis suggests that this growth will continue, fueled by supportive government policies and technological innovations. The projected market size will be impacted by factors like production costs, adoption rates of electric vehicles, and the development of novel battery chemistries.
Forecasting precise figures is complex, but various market research firms predict a substantial increase in the next decade, with electric vehicles as a key driver. For instance, the increasing popularity of electric scooters and bicycles will also contribute to battery market growth.
Emerging Trends and Future Possibilities
Several emerging trends are shaping the future of battery technology. Solid-state batteries, with their potential for higher energy density and improved safety, are a prominent example. Other innovations, such as lithium-sulfur batteries and lithium-air batteries, are under development, promising further advancements in energy storage capacity and efficiency. Furthermore, advancements in battery management systems are crucial for optimizing performance and safety.
These systems are becoming increasingly sophisticated, enabling better control and monitoring of battery health and performance. The integration of AI and machine learning into battery management systems could further enhance their capabilities.
Role of Government Policies and Regulations
Government policies and regulations play a critical role in shaping the future of battery technology. Incentives for electric vehicle adoption, investment in battery research and development, and regulations on battery safety are key factors influencing market growth and technological advancement. For example, mandates for electric vehicle sales and tax credits can significantly accelerate the adoption of electric vehicles, which in turn stimulates demand for batteries.
Stricter safety standards, while initially presenting challenges, ultimately contribute to public trust and market acceptance. These policies ensure responsible development and deployment of this transformative technology.
Societal Implications
The advancements in battery technology will have profound societal implications. The shift towards electric vehicles, powered by advanced batteries, could significantly reduce air pollution and greenhouse gas emissions, contributing to a healthier environment. Increased energy storage capacity will also enable greater reliance on renewable energy sources, fostering a more sustainable energy infrastructure. Furthermore, the widespread adoption of electric vehicles could create new job opportunities in manufacturing, research, and maintenance.
However, equitable access to these advancements and the potential for job displacement in traditional industries also need consideration. Careful planning and proactive measures will be necessary to ensure that the societal benefits of battery technology are shared broadly.
Illustrative Examples
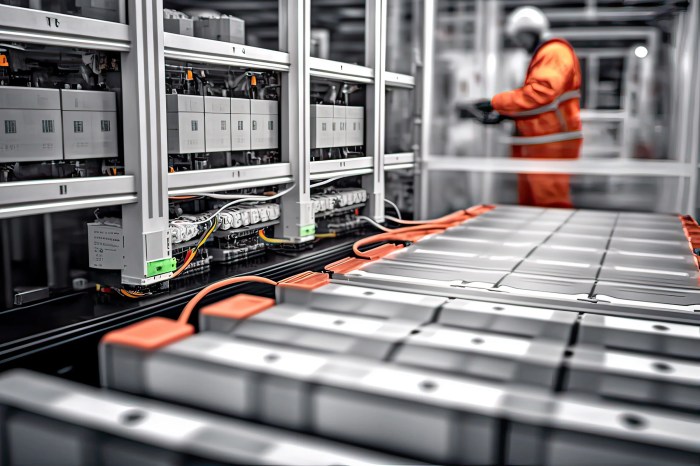
New battery technology breakthroughs are poised to revolutionize various industries, from electric vehicles to portable electronics. These advancements offer significant improvements in energy density, charging speed, and safety, leading to more efficient and reliable power solutions. The following examples highlight the diverse applications and potential impacts of these innovations.
Electric Vehicle (EV) Batteries
These advancements in battery technology are driving the growth of the electric vehicle (EV) market. Modern EV batteries, incorporating innovations in materials and cell design, provide greater energy storage capacity. This translates into increased driving range and reduced charging times.
- Enhanced Lithium-ion Batteries: Advanced lithium-ion battery chemistries, such as lithium-metal batteries, are being developed to improve energy density. This results in longer driving ranges and lighter vehicle weight. Imagine a Tesla Model S with a significantly extended driving range, without compromising performance or size.
- Solid-State Batteries: These batteries, featuring a solid electrolyte, promise faster charging rates and enhanced safety compared to traditional lithium-ion batteries. A hypothetical scenario involving a future electric SUV, featuring a solid-state battery, allows for a much faster charging time, reducing the downtime associated with charging.
- Battery Management Systems (BMS): Sophisticated BMS are crucial for optimizing battery performance and ensuring safety. Modern BMS systems can predict battery degradation, automatically adjust charging parameters, and enhance overall battery life. A robust BMS in a hybrid vehicle could effectively manage the flow of energy between the battery and the gasoline engine, potentially improving fuel efficiency and reducing emissions.
Portable Electronics
The increasing demand for portable devices with extended battery life is driving innovation in battery technology for consumer electronics.
- Miniaturized Batteries: Miniaturization is crucial for embedding batteries in increasingly compact devices. New technologies allow for the creation of smaller batteries with comparable or even greater energy density than their larger counterparts. Think about a smartphone with a longer battery life, despite a smaller form factor.
- Wireless Charging: Wireless charging technologies enable seamless power transfer without physical connectors. This enhances user experience and reduces the need for physical charging cables. Imagine a future where your laptop charges effortlessly when placed on a compatible charging pad.
Grid-Scale Energy Storage
Advancements in battery technology are crucial for managing fluctuating energy demands in the power grid.
- Large-Format Batteries: Large-format battery systems are essential for grid-scale energy storage. These systems can absorb excess energy from renewable sources and release it during periods of high demand. Consider a large-scale battery storage facility that can store solar energy and release it to the grid during peak hours, thus mitigating fluctuations in energy supply.
Table of Illustrative Examples
Application | Key Features | Benefits | Challenges |
---|---|---|---|
Electric Vehicles | Enhanced Lithium-ion, Solid-State, BMS | Increased range, faster charging, improved safety | Cost, manufacturing scalability, material sourcing |
Portable Electronics | Miniaturized Batteries, Wireless Charging | Extended battery life, convenient charging | Energy density limitations, safety concerns |
Grid-Scale Energy Storage | Large-format Batteries | Improved grid stability, renewable energy integration | High capital costs, battery lifespan |
Visual Representation (Conceptual)
Electric Vehicle Battery: A schematic diagram showing a lithium-ion battery pack, including individual cells, the battery management system (BMS), and cooling mechanisms. The diagram emphasizes the intricate connections and components within the battery pack, highlighting the crucial role of the BMS in monitoring and controlling the battery’s health.
Portable Electronic Battery: A cross-section of a small, high-density battery, highlighting the active materials and separator within the cell. This illustration emphasizes the compact design and sophisticated construction necessary to achieve high energy density in a miniature format.
Grid-Scale Battery System: A simplified diagram of a large-scale battery storage facility connected to the power grid. The diagram illustrates the flow of energy from renewable sources into the storage facility and back to the grid during periods of high demand.
Recent breakthroughs in new battery technology are seriously impressive. This progress is crucial for powering various devices, and understanding the intricacies of the underlying technology is key. For a deep dive into the specifics of managing and optimizing hardware, consider consulting Western Digital Technologies Driver A Comprehensive Guide, Western Digital Technologies Driver A Comprehensive Guide. Ultimately, these advancements in battery tech are paving the way for a more sustainable and efficient future.
Ending Remarks
In conclusion, the new battery technology breakthrough represents a significant leap forward in power storage. While challenges remain, the potential for transformative applications across diverse industries is immense. This innovation has the potential to revolutionize how we power our world, from electric vehicles to renewable energy solutions, and beyond.
FAQ Summary
What are the main types of new battery chemistries?
The major types include lithium-ion, solid-state, and flow batteries. Each has its own strengths and weaknesses in terms of energy density, power density, and lifespan.
What are the safety concerns associated with new battery technologies?
Safety is a crucial concern. Overheating, short circuits, and the handling of certain materials pose risks. Rigorous testing and safety measures are essential for responsible development and deployment.
How will new battery technology impact the transportation sector?
Electric vehicles and autonomous vehicles will greatly benefit from advancements in battery technology. Increased range, faster charging times, and lower costs will drive wider adoption.
What are some examples of real-world applications of this technology?
Portable electronics, renewable energy storage, and even aerospace applications are benefiting from these advancements. More specialized uses in marine and industrial contexts are also emerging.